Quantum Plasmonics
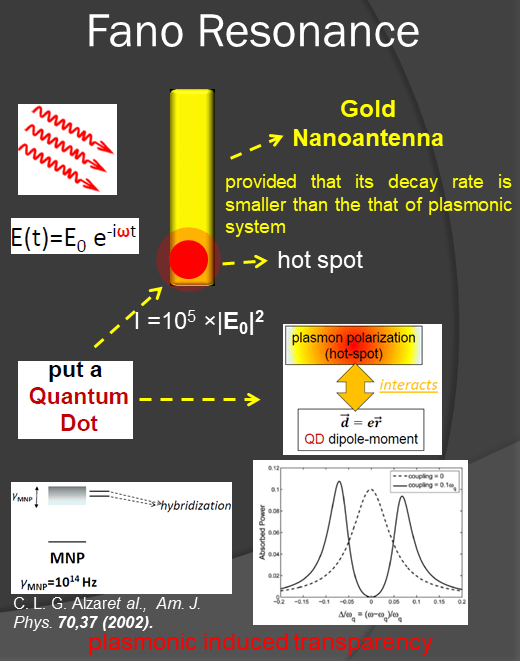
Quantum plasmonics have become one of the most influentional field in the recent years because of its tremendous implications in nonlinear optics and ultrafast quantum technology. The rapidly growing interest of the scientific community in plasmonics is mainly due to ability of metal nanostructures to confine light at the nanoscale far beyond the diffraction limit of classical optics. The main principle of the ultra-intense focused light (hotspot) around metallic nanoparticles consists of collective oscillations of free metal electrons, so-called plasmons upon interaction with resonant electromagnetic energy. This can be understood as special optical nanocavities which can mediate extraordinarily strong interaction of light with e.g. molecules. The theoretical description of the optical properties of such extremely small plasmonic nanocavities often surpasses the limits of classical physics and requires a quantum mechanical treatment. To that end our group participates in the development of the quantum mechanical description of plasmonic excitations as harmonic oscillations within the framework of Heisenberg equation. In close collaboration with the groups of Emre Taşgın and Alpan Bek in Ankara (Turkey), we address effects such as quantum interaction of nanostructures in nano-sized gaps, effects of local and non-local intense modes in the near and far field resonant coupling, coupling of intense localized plasmonic field to the quantum objects or semiconductor quantum dots driving the localization of light down to the nano scale. In our group we develop effective semi-classical models that can bridge the gap between the full quantum and purely classical descriptions of the electromagnetic response.
The interaction of surface plasmon resonance with molecular systems requires a study that deals with the quantization of the electromagnetic field associated with the plasmons in order to adequately address the coherences and quantum dynamics of the system. The quantization of a plasmonic field in cavity can be addressed through the quantum electrodynamics (QED). QED provides a suitable platform which incorporates with the quantum statistics of plasmonic and molecular excitations, as well as the interaction with the field reservoir. All of these aspects are required for an accurate description of quantum states, decay processes, or collective light emission from molecules.
One of the efforts of our group is to develop models to describe the coupling of quantized modes (plasmons) with molecules in (sub)nanometric cavities by using general QED methods to address phenomena such as fluctuation-driven spontaneous emission of molecules or surface-enhanced Raman scattering (SERS), in order to reveal novel non-classical processes and phenomena.
Quantum Electrodynamics (QED)
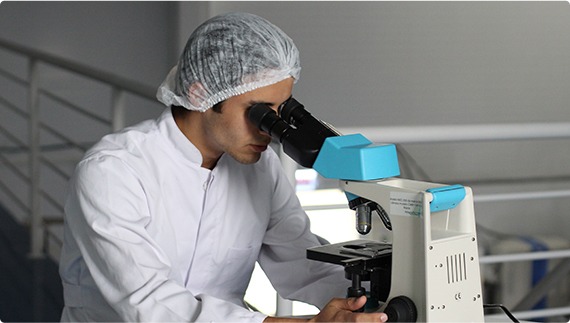
Metallic Nanoantennas
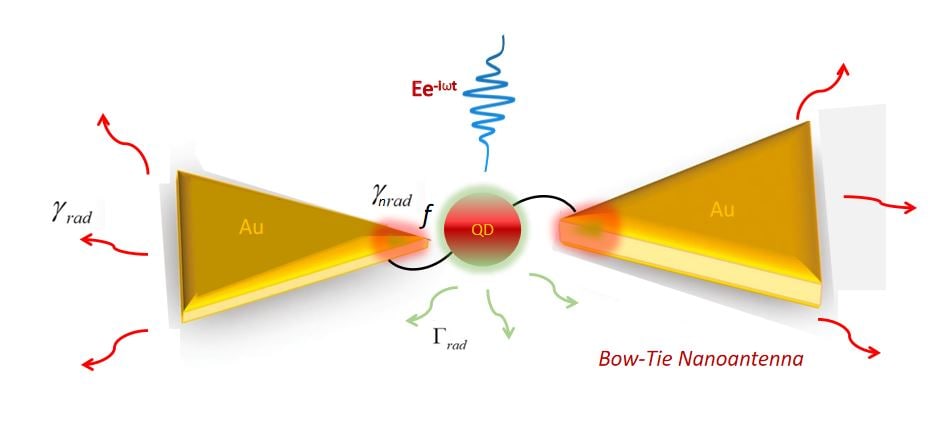
Plasmonic nanoparticles support surface plasmon resonances (SPRs), which are collective oscillations of the electronic charge density localized at the surface of metallic structures. The optical properties of these metallic nanostructures differ from the optical response of the same metallic material in bulk. Among many plasmonic nanostructures, metallic nanoantennas exhibit unique optical properties derived from the huge enhancement and confinement of the electromagnetic fields at nanoscale dimension associated with the excitation of localized surface plasmon resonances (LSPR). The LSPR properties of plasmonic nanoantennas are governed by three major structural designs: tunable dielectric-control nanostructures, nanoparticles with tunable gaps, and nanostructures self-tunable by charge carriers.
The energies of LSPRs also depend on the geometry of the nanantenna structure, as well as their sensitivity to the dielectric environment. Thus, depending on the particular property of interest and, by engineering the shape, the materials or the geometric configuration, a huge variety of nano antennas has been synthesized, such as nanorings, nanoparticle dimers, nanoshells, nanorods, or nanostars.
Plasmonic nanoantennas have played a significant role in enhancing the efficiency of optoelectronic and photonic devices. In a coupled linear and nonlinear environment these nanoantennas display enhanced characteristics features which enables them to employ as an effective tool for biological fingerprinting and biosensing technology. Nanoantennas have also become a potential source of confined light in nanolaser systems.
In conventional optics, the resolution of an optical instrument is restricted by the diffraction limit. However, metallic and dielectric nanostructures are able to localize the electromagnetic field into a length scales much smaller than the wavelength of light, thus permitting optical imaging beyond the diffraction limit. The strongly localized non-radiative electromagnetic field is termed as near-field, because it appears only close to the surface of nanostructures.
The advancement in scanning probe microscopy has lead to form a group of techniques referred to as near-field optical microscopies by exploiting the electromagnetic near-fields of nanostructures to get nanoscale resolution of objects. The standard approach to perform an apertureless near-field microscopy is to use a scanning tip to scatter the near-field of a nanostructure into the far-field, thus accessing the data on the scale, shape, and the dielectric environment of the nanostructure, etc. On the other hand, one could use of a scanning probe with an aperture, simultaneously illuminating the structure and collecting the scattered light.
We theoretically investigate the optical response of metallic nanostructures, optimize them to perform near-field microscopy, investigate the entire systems of nanostructures and their coupling to scattering probes.
Near-Field Optical Microscopy
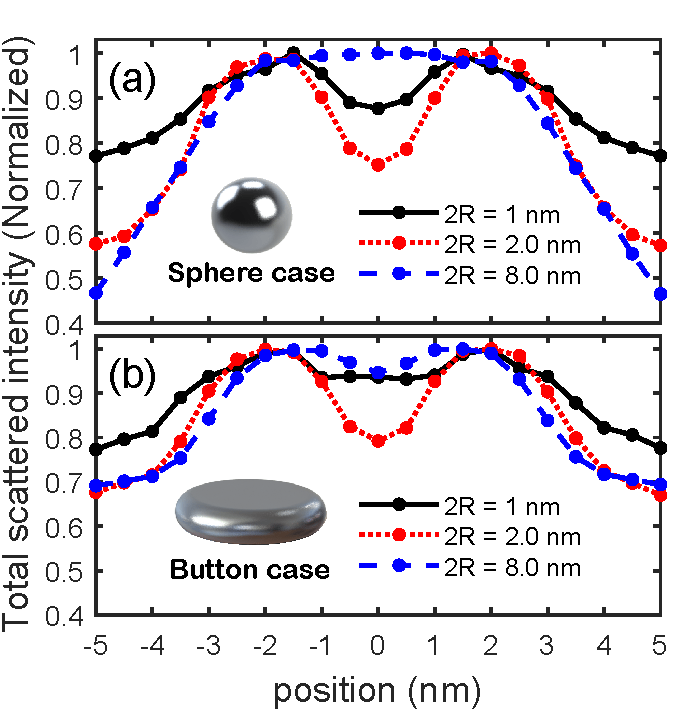
Surface/Tip Enhanced Raman Spectroscopy (SERS/TERS) with 2D materials
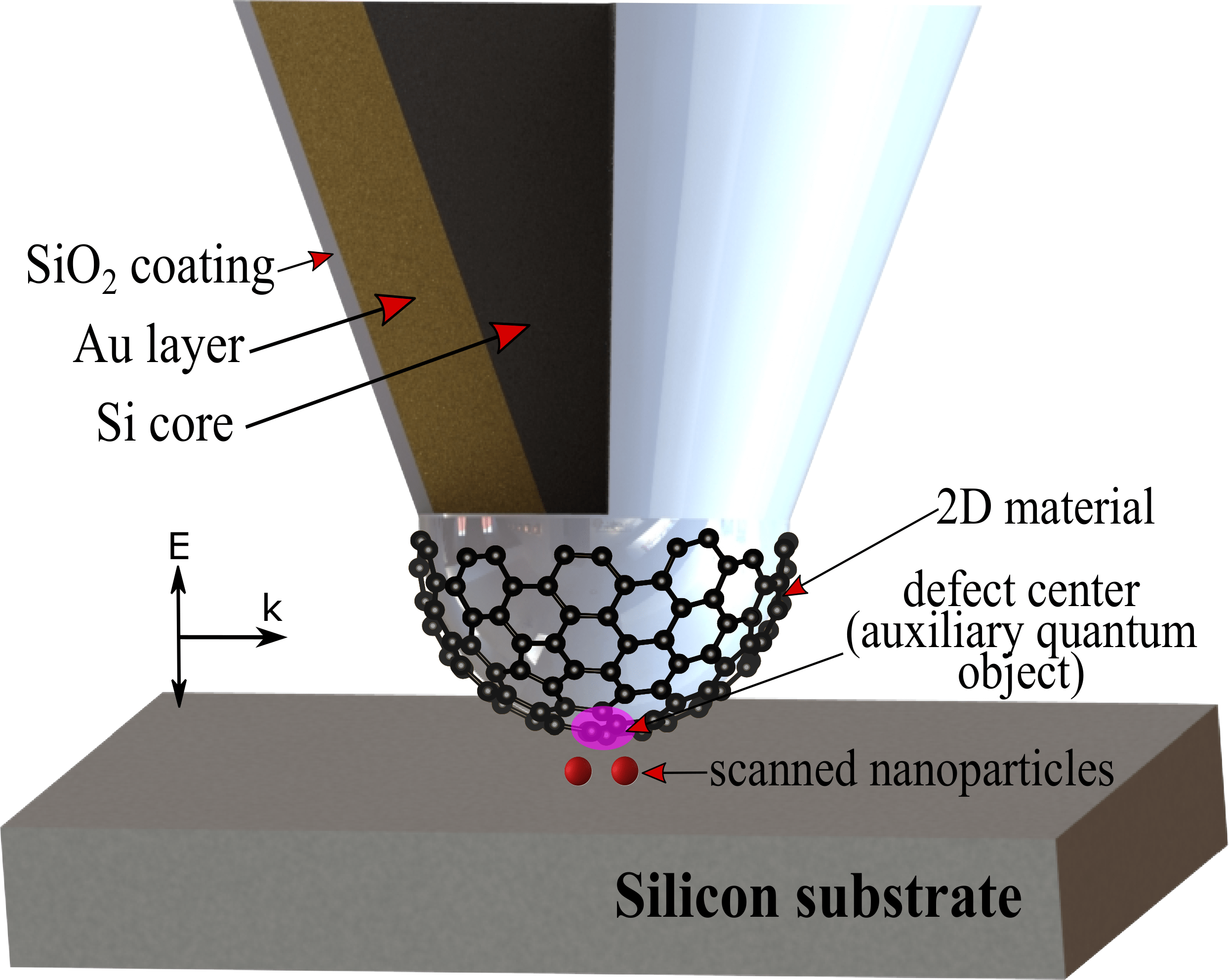
Metallic nanoparticles exhibit improved scattering and absorption properties at resonant electromagnetic energy while restricting electromagnetic fields in nanosize areas known as hot-spots. The highly concentrated electric field of hotspots can be employed to improve the interaction of light and matter at the nanoscale. Furthermore, the field localization and enhancement capabilities of metallic nanoparticles have been investigated in spectroscopy of extremely tiny molecules. This is known to as field enhanced spectroscopy. The tunability of the electromagnetic resonance of metallic nanoparticles with size, shape, and material composition allows access to molecular vibrations under a wide range of conditions.
Surface Enhanced Raman Spectroscopy (SERS) and Surface Enhanced Infrared Absorption Spectroscopy (SEIAS) are two well-known configurations for performing field enhanced spectroscopy. While SERS investigates enhanced electromagnetic scattering by molecules, mediated by the field boosting capability of metallic nanoparticles. On the other hand SEIRA focuses on enhanced electromagnetic radiation absorption by molecules.
We study theoretically the optical response of Refractory Transition Metal Nitride (RTMN) for optimizing them to perform field enhanced spectroscopy.